My research concentrates on topics in high-energy astrophysics with an emphasis on interpreting existing data and making predictions for future observations. My main interests are on the evolution of galaxies and their central supermassive black holes, and the physics of accretion disks around both black holes and neutron stars. My work often involves comparing computer based models with published data from X-ray, radio and/or infrared telescopes. All of these directions are guided by my underlying research philosophy of using any necessary tool or technique, unlimited by wavelength range or physical process, to make progress in understanding the relevant physics. Students working with me will be exposed to a wide range of astrophysical processes and theories, but will also learn to respect and be guided by observational constraints.
Are you a student interested in my research? Check out this FAQ Document which gives more information about working in my group. Please contact me if you have any questions or want to meet to discuss possible research projects.
Physics of Accretion Disks around Black Holes and Neutron Stars
Black Holes
Black holes are perhaps the most mysterious and enigmatic objects that one can imagine. Their gravitational fields are so strong that light is unable to escape their grasp, and even fundamental quantities such as space and time are severely disrupted by their presence. Yet, despite their fantastical nature, astronomers have compiled significant evidence that black holes are actually quite common and are lying at the centers of almost all massive galaxies. Moreover, black holes are now thought to have played a surprisingly important role in the evolution and formation of galaxies. Therefore, black holes are no longer the theoretical subjects of mathematical physicists; they are now known to be crucial to our understanding of how galaxies and other structures in the Universe formed and evolved. Elucidating the physical connection between black holes and the observable Universe is one the subjects of my theoretical research program.
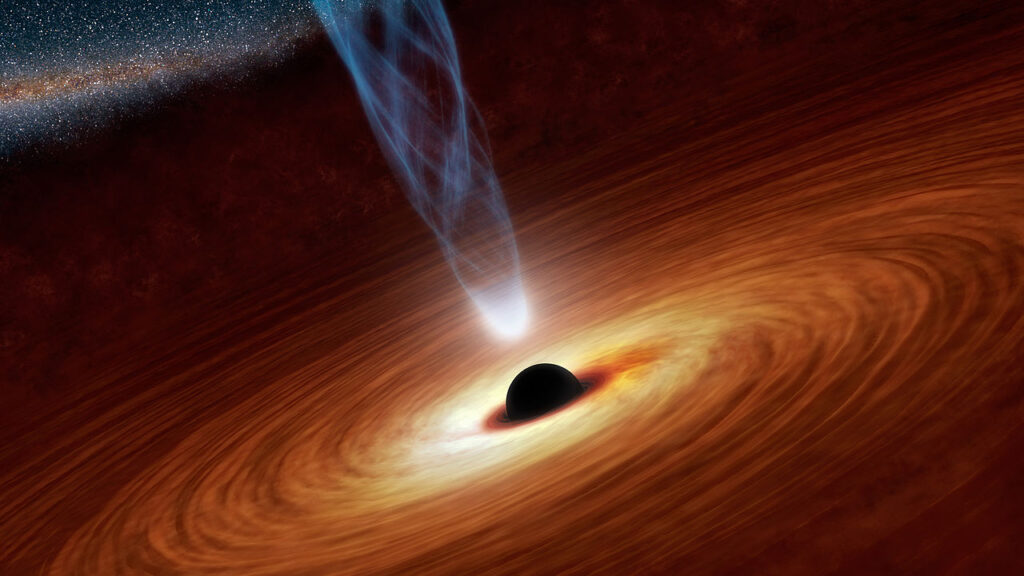
The gas and dust that falls toward a black hole in the center of a galaxy possesses a certain amount of angular momentum from its motion around the galactic center. Thus, just as a figure skater spins more rapidly when she pulls in her arms, the infalling material spins faster as it approaches the black hole. The incoming gas particles will collide and dissipate some energy before eventually settling onto an accretion disk. Viscous forces acting within the rapidly spinning disk remove energy and angular momentum from the material, which slowly spirals into the black hole. Accretion is one of the most complex and important problems in modern astrophysics and a complete treatment requires a combination of magnetohydrodynamics, radiative transfer and photoionization physics. In this area, my overall goal is to test accretion theories by determining the physical properties of accretion disks, and discovering how changes in their structure relate to different observational characteristics of accretion powered sources, such as the various manifestations of the AGN phenomenon.
To make progress, I use the features detected in the X-ray spectra of accreting supermassive black holes (known as active galactic nuclei, or AGNs), such as the soft excess and ionized reflection features, as a guide to calculate models of accretion disk structure and energy flows. These models are compared against the observational data to constrain the physical properties of disks close to the event horizon.
Neutron Stars
Thermonuclear explosions on the surface of neutron stars, generally known as Type I X-ray bursts, can be used to study the behavior of matter under conditions impossible to produce in a terrestrial experiment. Careful analysis of the burst spectrum and luminosity has been used to provide crucial constraints on the neutron star equation of state, one of the most important unsolved problems in high-energy astrophysics. In addition to their value for equation-of-state studies, it has recently been recognized that X-ray bursts are a powerful and unique probe of accretion disk physics, as the intense release of radiative energy in the burst over a short timescale (seconds for Type I bursts; hours for superbursts) significantly impacts the structure of both the disk and corona in ways that can be observationally measured. The location and spectrum of the primary X-ray source during a burst is well known and easily modeled, allowing for a relatively simple and repeatable experiment on the dynamics of the innermost regions of accretion disks. In fact, studying burst- disk interactions has the potential to reveal the inner workings of accretion flows in a way that cannot be replicated by any other accreting system.
The physical response of an accretion disk and corona to the impact of an X-ray burst is currently not well understood. A wide range of processes and responses are possible, including outflows (driven by radiation pressure), inflows (driven by Poynting-Robertson drag), and heating and cooling effects. All of these processes are directly related to the physical properties of the accretion disk (e.g., its surface density, scale height and effective viscosity), and therefore understanding and observationally measuring the interactions will lead to crucial insights into the workings and properties of accretion flows. I am collaborating with Prof. Chris Fragile (College of Charleston) on numerical simulations of an accretion disk subject to the sudden, intense radiation field of an X-ray burst. Our results, published in Nature Astronomy, found that Poynting-Robertson drag efficiently drains the inner accretion disk during the burst causing the inner edge of the disk to recede by several kilometers before recovering as the burst fades.
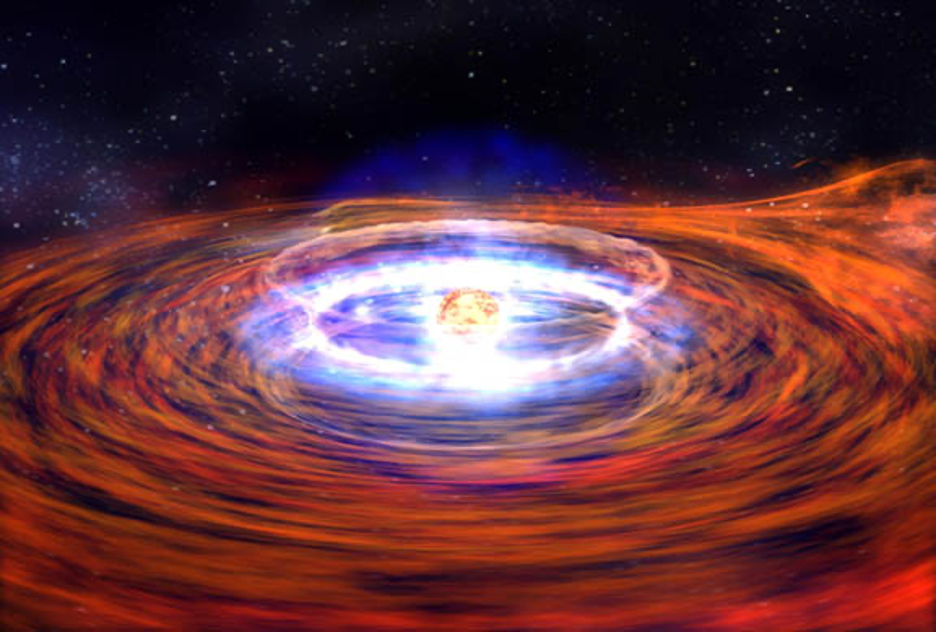
These results clearly demonstrate the impact of the burst on the disk, but further work is needed to understand how these interactions can be measured through observations of X-ray bursts. I continue to study the observational and theoretical consequences of X-ray bursts interacting with accretion disks.
Evolution of Active Galactic Nuclei
Astronomers have discovered that the centers of most galaxies, including the Milky Way, harbor a supermassive black hole, each with a mass that can be equal to a million or even a billion Suns. Despite its enormous mass, the gravitational influence of a black hole in a galaxy extends only about 30 light-years, which is tiny in comparison with the 100,000 light-year diameter of a typical galaxy. The vast majority of a galaxy’s stars, therefore, are immune to the presence of any black hole that may be lurking in the galactic center. Yet amazingly, astronomers find that the mass of a supermassive black hole is always about 0.1% of the host galaxy mass, independent of the size of the galaxy. This result indicates that the formation of galaxies and black holes must be synergistic with each formation mechanism impacting the other. A complete understanding of the black hole-galaxy connection is one of the foremost problems in modern astrophysics. My overall goal in this area is to understand the physical processes at work that lead to this combined evolution of supermassive black holes and galaxies.
In pursuit of this goal, my group focuses on the class of galaxies that host growing black holes, known as Active Galactic Nuclei (AGNs). These black holes are gaining mass by accreting material from their surroundings. This process releases tremendous amounts of energy in the form of radiation, making AGNs some of the most luminous objects in the Universe and detectable by telescopes back to the epoch of galaxy formation. Studying AGNs and their host galaxies therefore provides a window into the physics of the black hole-galaxy connection.
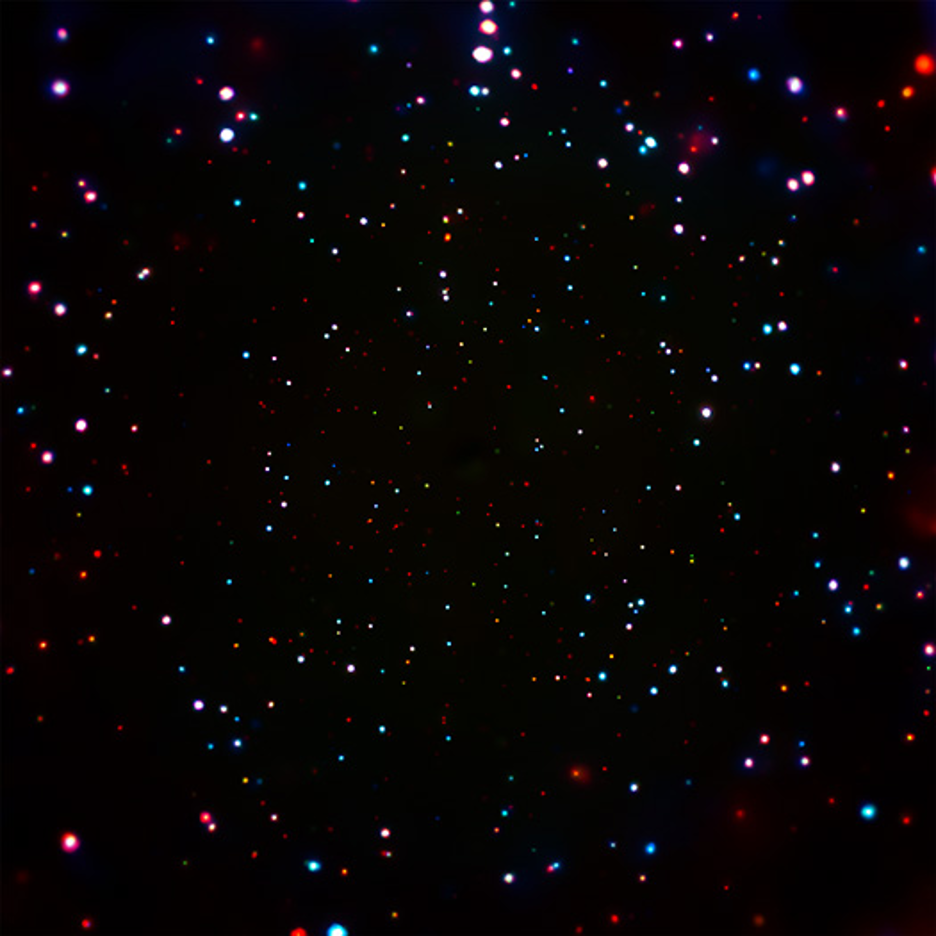
Over the next 5 years, my goal in this area is to produce an innovative new model of the X-ray Background (XRB; the integrated emission from all accreting black holes in the Universe). Unlike previous XRB models which have been based on phenomenological relationships, I intend to develop a model that is physics based; where, for example, luminosities are replaced by Eddington ratios, the X-ray power-law observed in AGNs is produced by Comptonization of accretion disk photons, and the X-ray reflection strength is proportional to the covering factor of Compton thick gas at parsec scales. The results of this effort will be crucial in interpreting the X-ray survey data produced from new missions such as eROSITA and Athena.